Potential of Controlled Traffic Farming for Enhanced Soil Health and Productivity
ID
BSE-374NP
Introduction: Farm Traffic
Over past decades, there has been a steep emergence in the use of larger and heavier agricultural machinery including for tillage, planting, spraying, and harvesting. Although this is done to achieve higher productivity and efficiency (Khanal, 2021), the unregulated traffic in the crop fields raise wheel loads to result in heavy soil compaction risks. Soil compaction is one of the major reasons for soil health degradation impacting physical, chemical, and biological properties of the soil and eventually the crop productivity. For example, unregulated heavy traffic in the field can lead to patches of poor crop performance, standing water, and risks of other biotic and abiotic stresses to the crop (Antille et al., 2019) (Fig. 1). This is because the soil compaction increases soil bulk density and decreases soil porosity, nutrient and water availability. On-farm research indicates that over 50-80% of the fields witnessed traffic in any given year when field traffic is not controlled (Antille et al., 2019; Galambošová et al., 2014). The effects of soil compaction, especially in the subsoil, are even more long-lasting and intensified with each machinery pass. It has been reported that on average, about 70% of the field can be trafficked in a conventional cropping system while the traffic could be up to 50% in the no till systems (Dyck, 2017). Moreover, about 70-80% of the soil compaction can occur on the first pass itself, indicating that driving even once can create enormous soil compaction problems. While most of the damage can be done in the first pass, the second and further passes will worsen soil health, potentially beyond recovery (Dyck, 2017). Natural soil recovery depends greatly on the soil type, compaction levels, amount of drying and wetting, and cycles of freezing and thawing. Despite this, for all soil types, the chances of soil normalization or recovery from compaction decreases with the depth (Antille et al., 2019). In a nutshell, soil compaction from unregulated machinery traffic can lead to loss in productivity and efficiency as well as endanger sustainable production due to irreversible soil health (Fig. 1). We present the impact of soil compaction on crop yield and assess Controlled traffic farming (CTF) as a possible solution.
Controlled Traffic Farming
Controlled traffic farming (CTF) has evolved as a method to minimize soil degradation due to compaction resulting from unregulated traffic of heavy machinery (Fig. 2). CTF confines all high load wheel/track traffic from equipment to permanent shared lanes in a field to minimize compaction and simplify farming operations. While not a new concept, the advent of GPS-controlled equipment and GIS software facilitates CTF adoption (Tamirat et al., 2022). Permanent traffic lanes are often used to mark common wheel ways, which are used for all field traffic. Although the permanent track area from CTF compacts the soil, about 70–80% of the farmland does not get compacted (Tamirat et al., 2022). CTF systems have the capability of maintaining structural integrity of soils in the no-traffic zones and save operation time (Antille et al., 2019).
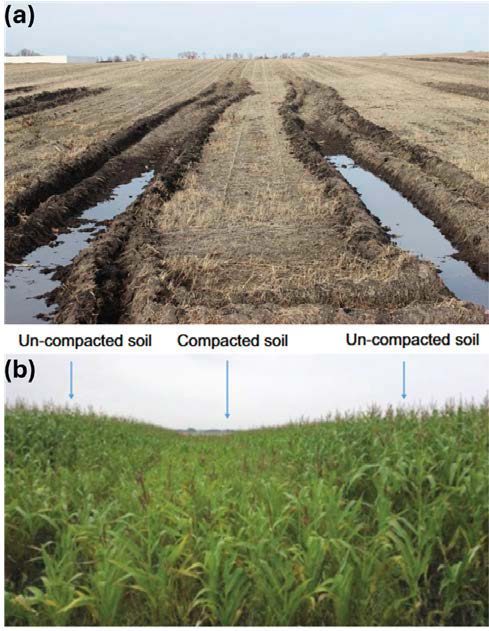
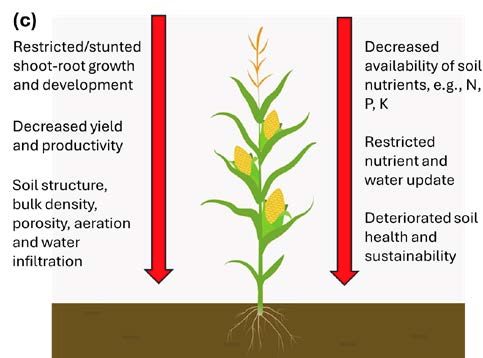
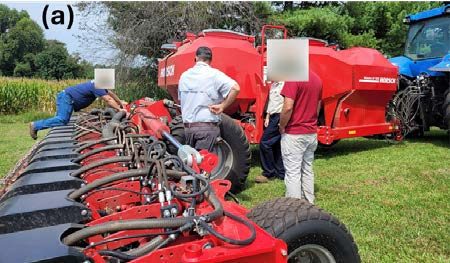
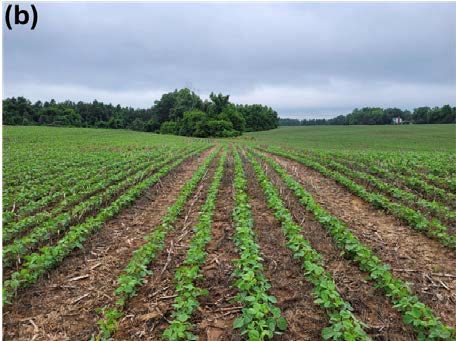
Experimental study
A study was conducted at two grower farms planted with corn and soybean in Blackstone, VA in 2023 to quantify the impacts of traffic on soil compaction and eventually the crop health and yield. These fields have been under CTF since 2018. Corn and soybean fields were divided into 12 blocks, 6 for cover crop (CC) and 6 for no-cover crop (NC), and each block marked for two traffic zones (no-track and track). Soil compaction measurements were collected in these blocks using a soil cone penetrometer (FieldScout SC 900) that read compaction down to 18-inch depth in soil. A total of 18 measurements were collected per block with 6 in the no-track zone and 12 in the track zone to quantify soil compaction. Soil compaction measurements were collected after planting and after harvest for corn, and after planting and before harvest for soybeans. The crop yield information for soybeans was gathered from the combine harvester and was used for impact evaluation of soil compaction. To assess the impact of soil compaction also on crop health, very high-resolution imagery data was collected from low-orbital satellites at 0.5 m/pixel resolution. The imagery data was processed in GIS software to derive Normalized Difference Vegetation Index (NDVI), an indicator of crop health and yield where higher value represents healthier crops and higher yield potentials.
Results
The near/after harvest compactions were evaluated as until that time, all major operations would have accumulated the impact on soil for that growing season. For the corn crop field, the average soil compaction pressure (SCP) increased down till the hardpan (0–10-inch depth) with track zone (374 kPa) having higher SCP than no-track zone (362 kPa) (Fig. 3a). After the hardpan, the track zone consistently had higher compaction than the no-track zone down to 18- inch depth (Fig. 3a). For the soybean crop, SCP in the track zone was consistently higher than the no-track zone till and beyond the hard pan (0–18 inch) (Fig. 3b). For example, at the 10-inch depth, the track zone SCP was 298 kPa compared to the no-track zone SCP of 281 kPa (Fig. 3b). The SCP due to CTF under no- cover-crop (NC) was slightly higher compared to the cover crop (CC) conditions in the corn field. While the SCP differences were not visible, for NC and CC in soybean field. The presence of cover crop poses some level of resistance to traffic weight unlike NC conditions. This creates a possibility of cover crops developing higher root biomass due to growth and penetration thereby breaking down compacted soil layers and retaining porosity. This is unavailable to soil under NC conditions and thereby remains more prone to compaction. SCP in the crop root zone at early growth stages (11–18-inch depth) certainly had a negative impact on root and shoot development, that impacted crop health (NDVI) later in the season (Fig. 5a). In addition, crop yield had a strong positive correlation with NDVI (Figure 5b, r = 0.63). These two observations suffice that soil compaction will have a negative impact on crop yield. An unregulated traffic field was not compared in this study.
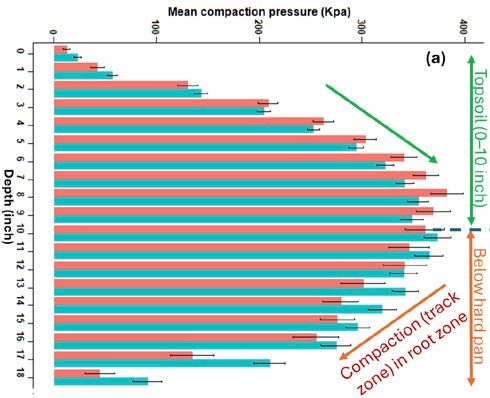
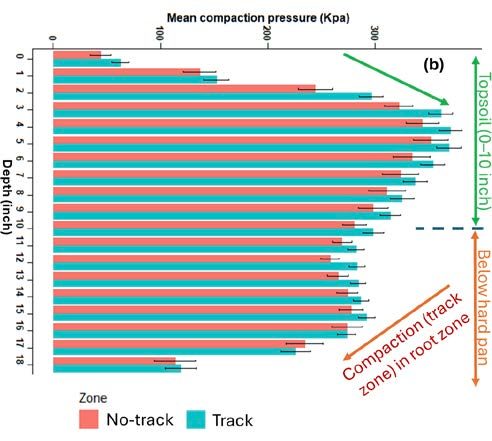
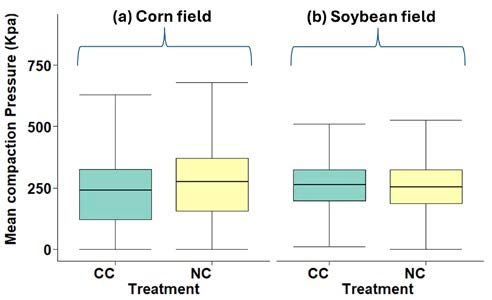
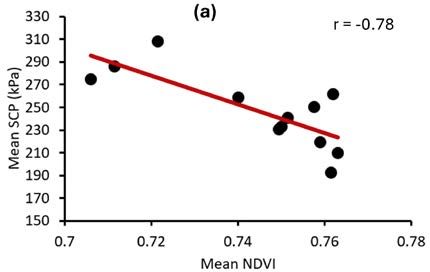
Conclusion
Study shows that the soil compaction was higher for the track zone compared to the no-track zone for both the corn and soybean growing fields. Importantly, this impact was prominent in the root zone depth i.e., till and beyond the hard pan. In some cases, the compaction varied so prominently that it led to the formation of two hard pans. Evaluations for the CTF also showed a trend that the field treatments with no cover crop experienced the highest soil compactions whereas the treatments with cover crops experienced the least compaction in most cases. It was also evident that soil compaction led to reduced crop health which likely also impacts the crop yield. Studies have reported earlier that it may be relatively less difficult for the soil above the hard pan to recover from compaction compared to depths beyond the hard pan. Most importantly, this is the depth that represents the crop root zone where roots expand and access aeration, nutrients, and water during early crop growth. Therefore, it becomes highly critical to minimize the soil compaction from unregulated traffic of heavy agricultural machinery. CTF can be a potential solution that limits the traffic to the smallest portion of the overall field thereby reducing soil compaction and enhancing productivity, efficiency, and environmental sustainability.
A major impediment to the adoption of CTF is getting all equipment at a common width, generally intervals of 30 or 40 ft wide (i.e. 30 ft planter and combine headers and a 90 ft wide sprayer). Having equipment widths that do share an interval would require major capital investments to adopt CTF but could be done over time as equipment purchases are made. Ideally, wheel spacing would also be the same between equipment, but a common equipment interval is a best fit setting for a CTF.
References
Antille, D.L., S. Peets, J. Galambošová, G.F. Botta, V. Rataj, M. Macak, J.N. Tullberg, W.C.T. Chamen, D.R. White, P.A. Misiewicz, P.R. Hargreaves, J.F. Bienvenido, and R.J. Godwin. 2019. "Review: Soil compaction and controlled traffic farming in arable and grass cropping systems." Agronomy Research 17(3): 653–682
Dyck, J. 2017. "Soil Compaction: Stay Off the Field Until the Soil is Ready." Fieldcrop News
Galambošová, J., M. Macak, V. Rataj, R.J. Godwin, M. Zitnak, B. Vitazkova, J. Dudak, and W.C.T. Chamen. 2014. "Yield performance of controlled traffic farming permanent tramlines." ASABE Annual International Meeting
Khanal, S. (2021). Assessing the impact of agricultural field traffic on corn grain yield using remote sensing and machine learning
Shaheb, M.R., R. Venkatesh, and S.A. Shearer. 2021. "A Review on the Effect of Soil Compaction and its Management for Sustainable Crop Production." Journal of Biosystems Engineering 46(4): 417–439
Tamirat, T.W., S.M. Pedersen, R.J. Farquharson, S. De Bruin, P.D. Forristal, C.G. Sørensen, D. Nuyttens, H.H. Pedersen, and M.N. Thomsen. 2022. "Controlled traffic farming and field traffic management: Perceptions of farmers groups from Northern and Western European countries." Soil and Tillage Research 217: 105288
Virginia Cooperative Extension materials are available for public use, reprint, or citation without further permission, provided the use includes credit to the author and to Virginia Cooperative Extension, Virginia Tech, and Virginia State University.
Virginia Cooperative Extension is a partnership of Virginia Tech, Virginia State University, the U.S. Department of Agriculture, and local governments. Its programs and employment are open to all, regardless of age, color, disability, sex (including pregnancy), gender, gender identity, gender expression, genetic information, ethnicity or national origin, political affiliation, race, religion, sexual orientation, or military status, or any other basis protected by law.
Publication Date
April 15, 2025